From the Desk of Gus Mueller
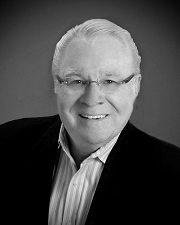
Okay, let’s start with a little quiz: What do London taxi drivers, orchestra conductors, second-language learners, and food-storing birds all have in common? You guessed it . . . research has shown that all four groups have experienced structural brain changes due to neuroplasticity. Neuroplasticity refers to the amazing ability of the brain to adapt to changes in an individual's environment and sensory inputs by forming new neural connections over time. It’s commonly discussed relative to recovery from traumatic brain injury, but also applies to a wide range of more subtle factors.
There is some very choice brain real estate located in the temporal lobe, so it’s pretty easy to see how neuroplasticity applies to hearing loss, and the results of subsequent treatment with hearing aids and cochlear implants. In general, there are two easy-to-remember themes: “use it or lose it” and “use it and improve it.”
Our 20Q guest author, Dr. Anu Sharma and her research team have shown that is possible to track hearing-related neuroplasticity through the use of the P1 cortical auditory evoked potential biomarker. Her group also has studied cross-modal reorganization using other electrophysiologic measures. You’ll have to read about it yourself, but some particularly interesting findings from this latter research relate to the need for well–fitted hearing aids. Who would have ever guessed that audibility is important!
Anu Sharma Ph.D. is Professor in the Department of Speech Language and Hearing and Fellow in the Institute for Cognitive Science and Center for Neuroscience at University of Colorado at Boulder. Her research is focused on examining neuroplasticity in pediatric and age-related hearing loss and has been funded by the United States National Institutes of Health for over two decades.
Most of you already know Dr. Sharma from her 100s of scientific publications and presentations. She is a frequent speaker at both national and international scientific conferences, and her research has been highlighted in a report to the US Congress by the National Institute for Deafness and Communication Disorders. She has been selected to give the Marion Downs Lecture at the American Academy of Audiology, and most recently, honored to deliver the prestigious Carhart Memorial Lecture at the 2022 American Auditory Society meeting.
Neuroplasticity is a fascinating area, and we’re fortunate to have people like Anu supplying us with important research findings. Whether you’re a London taxi driver, or a new hearing aid user, neuroplasticity can be your best friend!
Gus Mueller, PhD
Contributing Editor
Browse the complete collection of 20Q with Gus Mueller CEU articles at www.audiologyonline.com/20Q
20Q: Harnessing Neuroplasticity in Hearing Loss for Clinical Decisionmaking
Learning Outcomes
After reading this article, professionals will be able to:
- Describe neuroplasticity and how clinical audiologists can use this information in investigating and diagnosing hearing loss.
- Explain the use of the P1 cortical auditory evoked potential (CAEP) biomarker in determining candidacy for hearing aids and/or cochlear implants in children with hearing loss.
- Summarize the clinical implications of key research of the neuroplastic changes associated with deafness and hearing loss.
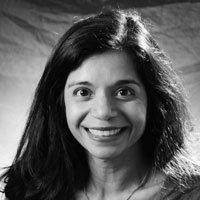
1. What is meant by neuroplasticity?
One of the most remarkable aspects of the brain is its ability to adapt in response to change. Neuroplasticity refers to the brain’s ability to respond to intrinsic or extrinsic stimuli by re-organizing its structure, function and connections. This includes changes in underlying neural connections, pathways, and networks which occur as a result of maturation, learning, sensory stimulation, sensory deprivation, injury and disease. Neuroplasticity is a process which occurs at all levels of neural pathways and throughout the entire lifespan.
2. Why is understanding neuroplasticity relevant for clinical audiologists?
As hearing care professionals—when we fit hearing aids, activate a cochlear implant, or guide rehabilitation for children or adults to help them reach optimal performance after intervention—all these processes are subserved by underlying neuroplastic changes. A better understanding of the cortical neuroplasticity that accompanies hearing loss may also allow us to incorporate improvements in the design and fitting of hearing prostheses to allow them to better accommodate altered cortical processing.
We believe that by harnessing cortical neuroplasticity for clinical use, we will be able to develop better tools (e.g.,, brain-based biomarkers) for investigating and diagnosing hearing loss. The same biomarkers will enable us to optimize intervention in individual patients by assessing how well the chosen intervention or rehabilitation is working. This approach is often referred to as customized or personalized medicine.
3. I really don’t know what you mean by a “biomarker?”
There are different possibilities, but over several decades in our laboratory, we have developed and refined the use of the P1 cortical auditory evoked potential (CAEP) biomarker. The P1 response is the first positive peak in the CAEP. The P1 response is present in infancy, occurring at a latency of 300 milliseconds post-stimulation. As a child grows and their auditory system becomes more efficient, the P1 response decreases systematically in latency until it reaches 50-70 milliseconds in the second decade of life. Because the P1 latency changes as a function of age, it can be used as an objective biomarker of cortical auditory maturation (Sharma et al., 2015).
We have collected and published normative data on the P1 response in childhood and established confidence intervals for normal development of this response. This allows us to compare individual pediatric patients with age-matched normally hearing children to determine if the patient’s auditory cortex is maturing typically, shows delayed or immature development, or shows atypical or abnormal development (Sharma et al., 2013; Cardon et al., 2012). The P1 biomarker can allow us to determine candidacy for hearing aids and/or cochlear implants in children with hearing loss who have additional physical, cognitive or developmental disabilities which make traditional behavioral testing difficult. The same P1 biomarker enables us to determine whether, and to what extent, cortical auditory development occurs after intervention with hearing aids or cochlear implants, a necessary occurrence for learning oral speech and language.
4. Can you provide some real-life clinical case examples that highlight the use of the P1 biomarker?
Sure, here are a couple cases, adapted from Cardon, Campbell and Sharma (2012), that describe how we have used the P1 biomarker in clinical decision-making in pediatric cases of Auditory Neuropathy Spectrum Disorder:
Case 1: This child failed his newborn hearing screening bilaterally and was diagnosed with ANSD before age 3 months. His birth history revealed use of supplemental oxygen. He was referred to our laboratory for P1 testing before and after intervention with bilateral hearing aids. Figure 1 (left panel top) depicts P1 CAEP waveforms prior to hearing-aid fitting. A robust and replicable P1 response was present; however, when plotted against 95% confidence intervals for normal P1 latency development, the child’s response was delayed for his age, indicating delayed cortical auditory maturation (Figure 1 right panel unfilled square). He was fitted with bilateral hearing aids when he was 7 months old. Figure 1b depicts the child’s P1 CAEP waveforms after several months of hearing aid use. Note that after intervention, a robust and replicable P1 response could be observed, and the latency of this response fell within normal limits for the child’s age (Figure 1 right panel filled square), consistent with the child’s progress in listening and spoken language after hearing aid fitting.
Figure 1. P1 CAEP waveforms (with replications) for an ANSD child prior to hearing aid use (left panel top) and several months after bilateral hearing aid use (left panel). Averaged P1 CAEP latencies in the unaided and aided conditions are plotted against 95% confidence intervals for normal P1 latency. Results indicate delayed P1 latency prior to hearing aid use. Within several months of hearing aid use, P1 latency was age-appropriate. Adapted from Cardon et al (2012). Figure republished from J Am Acad Audiol 23(5):396–411 with the permission of the American Academy of Audiology.
Case 2 was born premature, presented with several high-risk factors for hearing loss, including hyperbilirubinemia requiring blood transfusion, and prolonged mechanical ventilation while in the neonatal intensive care unit for a 7-week period. Absent ABR and present distortion product otoacoustic emissions (DPOAE) led to a diagnosis of ANSD before age 3 months. The child was fit with bilateral hearing aids at 3-4 months of age, and was referred for P1 testing in our laboratory due to concerns about significant delays in speech and language acquisition. Figure 2 (left panel top waveform) depicts P1 CAEP waveforms obtained with hearing aids. As can be seen, no replicable P1 response could be observed, indicating that the hearing aids were providing inadequate auditory input to allow for normal auditory cortical maturation. The P1 results were helpful in deciding on candidacy for cochlear implantation for this child.
Figure 2. P1 CAEP waveforms (with replications) for an ANSD child during bilateral hearing aid use (left panel top waveform) and several months after cochlear implantation of the left ear (left panel bottom waveform). Averaged P1 CAEP latencies hearing aid and cochlear implant conditions are plotted against 95% confidence intervals for normal P1 latency (right panel filled square). Results indicate absent P1 responses during hearing aid use. Within several months after left-ear cochlear implantation, P1 latency was age-appropriate (right panel filled triangle). Adapted from Cardon at al (2012). Figure republished from J Am Acad Audiol 23(5):396–411 with the permission of the American Academy of Audiology.
At age 1 year, 9 months, the child received a cochlear implant in the left ear. Figure 2 (left panel bottom waveform) shows the child’s P1 CAEP waveforms after several months of implant use. Cochlear implant results showed P1 latencies that fell within the 95% confidence intervals for the child’s age, indicating age-appropriate development of the central auditory pathways. In this case, electrical stimulation from the cochlear implant appeared to provide rapid improvement, leading to age-appropriate auditory cortical development.
The above cases highlight how the P1 biomarker may be used to determine what kind of intervention method may be best suited in difficult pediatric cases and to assess whether and to what extent the intervention is providing the necessary stimulation needed for the auditory cortex to develop as a precursor to speech and oral language acquisition.
5. With young children, how long does it take to see changes in the P1 biomarker after intervention?
Typically it takes about 3 months of wearing a well-fitted hearing aid or cochlear implant to see changes underlying neuroplastic changes in auditory cortical development.
6. Can the P1 biomarker be used to determine auditory thresholds in complex pediatric cases?
Auditory threshold estimation using the auditory brainstem response or auditory steady state response is often limited in some populations (e.g., individuals with ANSD or those who have difficulty remaining still during testing and cannot tolerate general anesthetic). However, cortical auditory evoked potentials (CAEPs) can be recorded in many such patients. For example, Cardon and Sharma (2021) explored whether the P1 CAEP (elicited by either a 1KHz tone or a speech sound /ba/) could be used to determine auditory thresholds in children who are typically developing and in children with ANSD. We found a close correspondence (within 10dB) between behavioral thresholds and CAEP thresholds. We also observed a clear pattern of CAEP amplitude decrease and latency increase as stimulus intensities declined until waveform components disappeared near auditory threshold levels.
Figure 3 shows the P1 CAEP elicited by the /ba/ sound as a function of intensity level for a group of 9 typically hearing children aged 4-6 years. A clear P1 response is seen at 10dBHL and is consistent with the average behavioral response of 8.89 dBHL.
Figure 3. CAEP grand average waveforms at descending intensities for children with normal hearing. Adapted from Cardon and Sharma (2021).
In Figure 4, we demonstrate the use of the P1 CAEP biomarker in obtaining thresholds in another child with ANSD. This child was born prematurely, required a twin-to-twin blood transfusion, mechanical ventilation, ototoxic medication, surgeries for necrotizing enterocolitis and spent several weeks in the neonatal intensive care unit (NICU). He suffered from hyperbilirubinemia which is a major risk factor for ANSD. Audiologic testing revealed an absent ABR with present cochlear microphonic and present OAEs consistent with a diagnosis of ANSD.
Case 3 was seen in our laboratory for P1 CAEP testing when she was 13 months old CAEPs were recorded to the /ba/ stimulus at 75, 65, 50, and 30 dBHL with the aim of estimating her auditory thresholds. The patient demonstrated robust and replicable CAEP responses at 75, 65, and 50 dBHL, but not at 30 dBHL. Thus, it was determined that his P1 CAEP threshold was at approximately 50 dBHL. Subsequent behavioral audiometry indicated that the thresholds were in the moderate hearing loss range (i.e., 45 and 43 dBHL PTA for the right and left ears, respectively), consistent with her P1 thresholds.
Figure 4. P1 CAEP biomarker threshold (50 dBHL) are consistent with behavioral audiometry thresholds (45 dBHL) for a young child with ANSD. Adapted from Cardon and Sharma (2021).
Children, such as those with ANSD and other complex medical histories, often present with absent or grossly abnormal ABRs and ASSRs, therefore, our ability to provide early intervention to these children is greatly limited. That is, beyond diagnosis, there are no physiologic measures of the auditory system that provide clinical information for diagnostically useful thresholds and the fitting of hearing aids. As we have shown here, the P1 CAEP biomarker can be used clinically to facilitate early intervention in this population.
7. Can this testing be conducted using standard ABR equipment, and are we able to bill for it?
We have successfully recorded P1 CAEPs using clinical equipment available in audiology clinics. We have described our methods for recording the P1 CAEP in detail in several publications (Campbell, Cardon and Sharma 2011; Cardon, Campbell and Sharma 2012). It is possible to bill for P1 testing using appropriate CPT codes. We also write a clinical report describing the results of the P1 biomarker for every patient we test.
8. What are some other kinds of neuroplastic changes that are associated with hearing loss?
Another interesting form of neuroplasticity that we see is called cross-modal neuroplasticity. When auditory cortical areas of the brain are deprived of normal input (i.e., the incoming sound is degraded and/or diminished), areas of the brain which normally process sound are “repurposed” by other intact modalities. Visual and somatosensory modalities repurpose auditory anatomy for their own processing.
9. What’s an example of this related to hearing loss?
Cross-modal neuroplasticity by both vision and somatosensation has been described in deaf children fitted with cochlear implants (CI). For example, Julia Campbell and I (2016) examined cortical visual evoked potentials (CVEPs) in response to an apparent motion stimulus in a group of CI children and age-matched normal hearing children utilizing high density 128-channel EEG. EEG source analysis via current density source reconstruction (CDR) was computed to localize sources of cortical activity. While visual stimulation in the normal hearing children expectedly localized to occipital (visual) cortical regions (Figure 5 upper left panel), visual stimulation in the CI children was localized to occipital (visual) cortical regions as well as temporal (auditory) cortical regions (inferior, middle, and superior temporal gyrus) (Figure 5 upper right panel), suggestive of cross-modal recruitment by vision. Earlier CVEP latencies (considered a marker of cross-modal plasticity), were correlated with worse scores on the BKB-SIN clinical test of speech recognition.
Cross-modal plasticity by the somatosensory system has also been documented in pediatric deafness in a study from our laboratory. Garrett Cardon and I (2019) recorded cortical somatosensory evoked potentials (CSSEP) using 128-channel high-density EEG in response to a vibrotactile stimulus delivered to the index finger in a group of deaf children fitted with CI, and in a group of age-matched normal hearing children. EEG source analysis using current density source reconstruction (CDR) showed that while the group of normal-hearing children exhibited cortical activity localized to brain regions typically associated with vibrotactile processing in the parietal cortex (e.g. pre/post central gyrus) (Figure 5 lower left panel), the group of CI children exhibited cortical activity localized in parietal cortex as well as temporal (auditory) cortex (e.g. inferior temporal gyrus, middle temporal gyrus, superior temporal gyrus) (Figure 5 lower right panel), suggestive of cross-modal re-organization by the somatosensory modality. Furthermore, greater cross-modal plasticity (as evidenced by earlier latencies of the CSSEP) was associated with worse speech recognition in noise on the BKB-SIN. Thus, both the visual and somatosensory modalities recruit auditory cortical areas in deafness, and this kind of cortical re-organization appears to be negatively associated with speech recognition ability. Overall, our results indicate that cross-modal recruitment reflects compensatory neuroplasticity by the visual and somatosensory modalities to help parse the degraded speech input to persons with hearing loss.
Figure 5. Current density reconstructions demonstrate expected occipital and somatosensory cortical activation (left upper and lower panels) to visual and vibrotactile stimuli respectively. Children with CI show additional activation of auditory cortical areas in response to visual (upper right panel) and somatosensory (lower right panel) stimulation suggestive of cross-modal re-organization from those modalities respectively (adapted from Campbell and Sharma 2016; Cardon and Sharma 2019). Source: Author.
10. What are your thoughts on neuroplasticity in our everyday patients—older adults with early-stage mild-moderate hearing loss?
We recently completed a research project that addressed that very question (Glick and Sharma, 2020). Hannah Glick and I examined neurocognitive changes in a group of 28 older adults (mean age 65.4 years) with early-stage, mild-moderate age-related hearing loss (ARHL) (average PTA = 27.1 dB HL) and 13 age-matched control subjects with normal hearing (NH). Many of the adults in the hearing loss group were unaware they had hearing loss prior to participating in the study, and people in both groups had no history of documented neurocognitive impairment. Participants in the ARHL group had not worn hearing aids previously. We collected baseline CVEPs from both groups in response to a visual motion stimulus (soon after hearing aid fitting) and 6 months post-hearing aid fitting. We also tested speech recognition in noise using the QuickSIN, and also tested cognitive function in both groups. Our cognitive test battery evaluated a variety of cognitive domains, including global cognitive function (using the Montreal Cognitive Assessment [MoCA]), executive function (using the Behavioral Dys-control Scale 2 [BDS-2]), processing speed (using the Symbol Digit Modalities Test [SDMT]), visual working memory (using the Reading Span Test [Reading Span]) and auditory working memory (using the Word Auditory Recall Response Measure [WARRM]). We assessed baseline cognitive function acutely aided in the hearing loss group to negate the possible effects of reduced audibility on cognitive test performance (all ARHL participants were fit with the same brand of hearing aids within ±5 dB of NAL-NL2 prescriptive targets from 250-4000 Hz).
11. So did you see any neuroplastic changes in the group that had hearing loss?
At baseline, the normal hearing group of older adult showed expected activation of visual processing regions of the brain in response to the visual stimulus. The untreated ARHL group exhibited visual activation plus additional activation of auditory regions of the brain, suggestive of cross-modal re-organization. In addition, the ARHL group showed recruitment of the frontal cortex during this task. Recruitment of the frontal cortex has been observed in participants with ARRHL and may reflect increased effort or cognitive load. Together, these findings indicate extensive cross-modal reorganization in the hearing loss group—a surprising finding given that many of the participants in this group were unaware they had hearing loss.
12. Did this age-related hearing loss impact cognitive performance?
When compared to the NH group, the untreated ARHL group exhibited significantly worse speech recognition in noise and poorer cognitive function across all domains assessed (global cognitive function, executive function, processing speed, visual working memory, and auditory working memory). Of note, greater cross-modal re-organization by vision (earlier CVEP latencies) was associated with poorer QuickSIN performance and more high frequency hearing loss (high frequency PTA). Further, cross-modal reorganization was associated with poorer cognitive functioning at baseline.
13. Is there any research indicating how quickly neuroplasticity changes occur after age-related hearing loss onset?
In a case study in our lab of an older individual with sudden sensorineural hearing loss, we examined cross-modal re-organization immediately following the onset of the hearing loss, 3 months, and 1 year after the onset. At the onset of hearing loss, cross-modal re-organization was not present in response to a visual stimulus. By 3 months after the hearing loss, there was clear evidence of activation of the temporal lobe in response to a visual stimulus (Glick and Sharma 2017). This cross-modal re-organization continued to progress as evidenced 1 year post hearing loss. While 3 months seems to be a likely time window after which neuroplastic changes will be apparent, more research is needed to fully answer this question, and given that our work presented here is a case study, these results must be interpreted cautiously.
14. What were the effects of treatment with amplification on cross-modal neuroplasticity?
Going back to the study I mentioned earlier, the ARHL participants were fitted with state-of-the art hearing aids at baseline using prescriptive targets. They were provided with counseling regarding how to use the hearing aids and were instructed to wear the hearing aids for 6 months (minimum of 5 hours/day) to remain in the study. Participants returned for interim hearing aid checks and routine data logging over the course of 6 months. Of the 28 ARHL participants initially enrolled, 21 met minimum daily use requirements and returned for 6-month follow-up testing. Baseline versus 6-month follow-up CVEP results for the ARHL group are summarized in Figure 6. At baseline, analysis of the CVEPs in the ARHL group demonstrated extensive cross-modal recruitment by visual stimuli of auditory cortical and frontal cortical regions (Figure 6 left panel). Six months later, we observed a reversal in cross-modal reorganization, such that there was a reduction in temporal and frontal cortex activity, and restoration of more typical visual processing patterns in response to visual activation (Figure 6 right panel).
Figure 6. (Left panel) At baseline, the ARHL group shows evidence of cross-modal re-organization, as evidenced by recruitment of temporal cortex and frontal cortex in addition to occipital regions typically associated with visual motion processing. (Right panel) At 6-month post-treatment with amplification follow-up, there is a reduction in temporal and frontal cortex activity and restoration of more typical visual processing patterns. Adapted from Glick and Sharma (2020). Source: Author.
15. Did the 6 months of hearing aid use also result in improvement in cognitive performance?
Accompanied by above-mentioned changes in neuroplasticity, we also observed significant improvements in the QuickSIN scores and cognitive function at 6-month follow-up. As shown in Table 1, significant improvements in cognitive function were observed in the domains of global cognitive function, processing speed, executive function, and visual working memory. There was no significant improvement in auditory working memory post-treatment with hearing aid use, suggesting that despite amplification, auditory working memory is required for helping parse degraded auditory input in hearing loss. Taken together, these results provide evidence that hearing aid treatment can provide neurocognitive benefit, at least in this group of adults with early-stage, mild-moderate hearing loss.
Table 1. Average speech perception in noise scores and cognitive performance between ARHL group (n=21) at baseline and 6-months post-treatment with hearing aids (Glick & Sharma, 2020). The ARHL group demonstrated significant improvements in speech perception in noise performance and cognitive function across the domains of global cognitive function, executive function, processing speed, visual working memory.
16. What are the implications of this study for clinical practices?
When hearing aids are fit, we should be using best practices. Probe-microphone measurements should be used to verify the hearing aid fitting. In an unpublished study of ours, neuroimaging evidence from long-term hearing aid users who were ‘underfit’ showed persistent cross-modal re-organization even after extended periods of hearing aid use. This suggests that correctly fitted hearing aids are important for providing the necessary gain to the auditory cortex to reverse cross-modal changes. Some clinicians delay recommendation of hearing aids until the hearing loss begins to affect communication, and many patients delay seeking intervention for their hearing loss until it has deteriorated communication and quality of life. However, based on our emerging evidence, hearing care professionals should educate patients about the effects of mild hearing loss on the brain and potential benefits of earlier treatment.
17. Is there a bio-marker we can use in the clinic to determine cross-modal re-organization in adults with ARHL?
Unlike the P1 CAEP biomarker in children, we have not yet developed a brain-based biomarker for cortical cross-modal re-organization that can be recorded using standard clinical audiology equipment. But we are working hard on this project. Interestingly, our study showed that adults who showed more extensive cross-modal re-organization by vision before hearing aid fitting tended to have worse speech perception in noise outcomes after hearing aid fitting. And remember we used the QuickSIN to measure speech perception in noise, a test which can be performed clinically.
18. Should clinicians be testing speech recognition in noise routinely for patients with ARHL?
One of the important findings from this study is the correlation between cross-modal re-organization and speech recognition in noise scores on the QuickSIN. Notably, poorer QuickSIN scores were associated with more extensive brain re-organization. While further research is needed, these results highlight the need for audiologists to look “beyond the audiogram” when considering the need for amplification. Routine use of the QuickSIN among audiologists has been reported to be as low as 10%. The QuickSIN test takes less than 5 minutes to administer and provides real-world pragmatic information about a patient’s speech intelligibility in noise.
19. You mention cognitive tests. Is that something that I should be doing?
There are some audiologists who conduct cognitive screening. Before initiating this screening, however, we recommend that clinicians develop strong referral networks with physicians and neuropsychologists so that patients who do need to be referred following a screening can readily access additional information, care, and support from a medical provider. While more research is needed, cognitive screening may also emerge as a secondary or tertiary outcome measure to assess benefit from treatment with hearing aids or cochlear implants, along with subjective questionnaires and speech-in-noise testing. While future research exploring the feasibility of cognitive screening in the clinic is needed, screening of co-morbid health conditions associated with ARHL (e.g., cognition, social isolation) may be within the scope of practice of a licensed audiologist. While many audiologists might feel uneasy about performing cognitive screening, it may be useful to consider investing in a training opportunity. For example, certification courses are available to learn how to administer the Montreal Cognitive Assessment (MoCA), a 5-minute, commonly used clinical training tool.
20. Is there a single take-home message that you can offer clinicians based on the summary of your research in pediatric and age-related hearing loss?
If I had to summarize the clinical implications of our research into the neuroplastic changes associated with deafness and hearing loss, it would be that early intervention with appropriate and well-fitted amplification and/or electric stimulation is necessary in both adults and children if we are to reverse compensatory neuroplastic changes and improve cognitive performance. As a field, we have successfully actualized the message of early intervention in pediatric hearing loss through newborn hearing screening and Early Hearing Detection and Intervention (EHDI) programs. However, we find that many audiologists and patients delay the use of hearing aids in adults with ARHL until hearing loss negatively impacts communication and quality of life. Our research suggests that even early-stage, mild-moderate hearing loss results in structural and functional brain changes and cognitive deficits. Even though our ARHL group had only early-stage hearing loss, post-treatment self-reported benefit and satisfaction rates were excellent. This, coupled with objective benefit from amplification (neurocognitive and speech perception benefit), presents a compelling case for earlier treatment of ARHL.
Editor’s Bonus Question: Anu, what a great review! I know we have some readers who are interested in conducting the testing that you have described. Could you maybe give us a brief description of your methods for recording the P1 CAEP?
I’d be happy to, Gus. We have published some detailed methods in other publications, which also might be helpful (e.g., Campbell, Cardon and Sharma 2011; Sharma, Nash and Dorman, 2009; Cardon, Campbell and Sharma 2012).
Typically, we record the P1 CAEP in response to a synthesized speech syllable /ba/, which has a total duration of 90 milliseconds and is presented at an interstimulus interval of 610 milliseconds. Noise bursts or pure tones also may be used to elicit the P1 response; however, it is important to consider the frequency spectrum of the stimuli in conjunction with the patient’s degree and configuration of hearing loss when using novel stimuli. We typically present the stimulus via loudspeakers placed at an angle of 45 degrees to the ear of interest. For bilateral responses, presentation occurs via a speaker placed at an angle of 45 degrees to each ear. Hearing aids and cochlear implants are set to the usual settings. The stimulus is presented at a comfortable loudness level, unless the subject required a higher level of intensity due to the severity of auditory thresholds. In all cases, every effort is made to ensure that stimulus presentation levels are made suprathreshold by at least 20 dB, and audibility of the stimuli is ascertained through any available audiological records and/or behavioral observation of the subject. We also present the stimulus via insert earphones if needed.
Adult patients and older children are seated by themselves. Younger children are typically seated on their parent’s lap in a comfortable reclining chair in a sound booth. Children are typically able to watch a movie on a monitor placed directly in front of the chair in the sound booth. Audio levels for the movie are muted.
We typically use silver/silver chloride cup electrodes for recordings, with the active electrode placed at Cz and the reference placed on one mastoid. Eyeblinks are monitored using a bipolar electrode montage (lateral outer canthus referenced to superior orbit), with eyeblinks rejected online during the test session. When performing clinical P1 testing, it is imperative to monitor for eyeblinks and ensure this artifact does not contaminate the recording. For children who received cochlear implants, multiple electrodes in addition to the Cz and mastoid sites are arranged across the forehead to minimize the artifact recorded on the scalp from the cochlear implants (Gilley et al., 2006). The recording window includes a 100- millisecond prestimulus and a 600-millisecond poststimulus time, and the sampling rate is set at 1.0 kHz. Responses are filtered from 0.1 Hz to 100 Hz. Additional artifact rejection is performed offline at a threshold of +/-100 mV. Two or more runs of at least 200 sweeps each are collected for each subject to determine waveform replicability, then grand-averaged. The peak of the P1 response is computed for each child and compared our normative data (i.e., the 95% confidence intervals for normal development of the P1 response).
Interpretation of the Waveforms: Assessment of the P1 peak in the CAEP response is performed by an audiologist experienced in electrophysiologic testing. Low-pass filtering (30 Hz at 12 dB/octave) is performed only after the P1 peak is ascertained, and then only for use in data presentation. In our experience, we have found that care must be taken when low-pass filtering or ‘‘smoothing’’ the CAEP waveform, as this transform may alter the appearance of the CAEP resulting in P1 responses that are inaccurately picked. For example, if artifact, such as that generated by a cochlear implant during CAEP recordings, is low-pass filtered, it may closely resemble a P1 response that does not actually exist. Furthermore, consideration of the ages of our subjects in waveform interpretation is of great importance as the morphology of the CAEP waveform changes as age increases. In older children and adolescents (7 to 11 years), the P1 bifurcates as the N1 develops, creating a P1- N1-P2 complex. To the untrained observer, the N1 of an older child may resemble a deprivation negativity which is seen in children who have an unstimulated auditory pathway. Thus, it is necessary for those interested in conducting P1 testing to become familiar not only with CAEP morphology in normal-hearing and hearing-impaired children, but with developmental effects resulting in morphological changes on the CAEP waveform. We have described several P1 morphological patterns, including the deprivation negativity, that we have observed in clinical populations in previous publications (e.g., Sharma, Nash and Dorman, 2009).
Minimization of Artifact: Recordings of cochlear implant artifact, as well as the inclusion of unwanted eyeblinks, can cause serious misinterpretation in determining the P1 peak response. The aforementioned method of online eyeblink removal followed by artifact rejection offline typically produces CAEP waveforms free of eye-related myogenic artifact. However, it is also helpful to monitor the patient’s ongoing EEG during P1 testing to ensure that any eyeblinks are indeed rejected. In order to monitor and reject eyeblinks a two-channel EEG system is needed at the very least. Of more serious concern is the cochlear implant artifact generated in CAEP recordings. When acoustic energy is transduced into electrical stimulation by a cochlear implant, an electrical field is generated over much of the scalp. This artifact may then contaminate and obscure possible P1 peaks in CAEP recordings, confounding interpretation. Our laboratory has dealt with the issue of cochlear implant artifact for several years and has found specific methods that have proven effective in obtaining artifact free P1 recordings in cochlear implant patients (Gilley et al., 2006). The method of greatest clinical use is the placement of multiple electrodes along the forehead in addition to the Cz and mastoid sites. This technique allows for a greater number of recording sites that may lie outside of the cochlear implant artifact field. However, the generation of artifact varies from patient to patient, making it nearly impossible to predict the optimal electrode site of recording. When performing P1 testing in a patient with cochlear implants, identification and subsequent rejection of the artifactual recording is a priority. In order to use the multiple electrode method for minimizing the cochlear implant artifact (described above and in detail in Gilley et al., 2006), a multichannel EEG recording system is needed.
High Density EEG recordings: In our laboratory, we use 128-channel, high-density EEG to evaluate how hearing loss affects the auditory cortex using cortical sensory evoked potentials. For example, we present sound (e.g, speech), visual stimuli, and/or vibrotactile stimuli to participants with normal hearing and participants with hearing loss, and we measure neural activity in response to these stimuli. These recordings are referred to as cortical auditory evoked potentials (CAEP), cortical visual evoked potentials (CVEP), and cortical somatosensory evoked potentials (CSSEP), respectively. Similar to auditory brainstem response testing, we evaluate CAEP, CVEP, and CSSEP waveforms in terms of their peak latencies and amplitudes. Because we are collecting data using many channels and topographically located electrodes, we can estimate where in the brain these responses originate from through an advanced statistical modeling approach called Current Density Source Reconstruction (CDR). CDR can be projected onto a 3D image of the brain (aka, structural MRI). While EEG lacks the fine spatial resolution of other neuroimaging methods, such as functional magnetic resonance imaging (fMRI) or positron emission tomography (PET), its superb temporal resolution (timing) and inexpensive and non-invasive nature makes it an excellent method to assess neuroplasticity in clinical populations with hearing loss.
Acknowledgments: I would like to acknowledge the students in my laboratory without whom these studies would not have been possible. All my doctoral students, past and present, have contributed significantly to our clinical studies on the P1 biomarker. Julia Campbell Ph.D. took the lead on the studies of visual cross-modal neuroplasticity. Garrett Cardon Ph.D. made possible the studies on somatosensory cross-modal recruitment. Hannah Glick Ph.D. led the research on the effects of amplification on neurocognitive plasticity. I would like to acknowledge the National Institutes of Health and the Hearing Industry Research Consortium for research funding.
References
Campbell, J. D., Cardon, G., & Sharma, A. (2011). Clinical application of the P1 cortical auditory evoked potential biomarker in children with sensorineural hearing loss and auditory neuropathy spectrum disorder. Seminars in Hearing, 32(2), 147-155.
Campbell, J. & Sharma, A. (2016). Visual cross-modal re-organization in children with cochlear implants. PloS One, 11(1). doi10.1371/journal.pone.0147793
Cardon, G., Campbell, J., & Sharma, A. (2012). Plasticity in the developing auditory cortex: Evidence from children with sensorineural hearing loss and Auditory Neuropathy Spectrum Disorder. Journal of the American Academy of Audiology, 23(6), 396-411.
Cardon, G. & Sharma, A. (2019). Somatosensory cross-modal re-organization in children with cochlear implants. Frontiers in Human Neuroscience, 13, 469. https://doi.org/10.3389/fnins.2019.00469
Cardon, G., & Sharma, A. (2021). Cortical neurophysiologic correlates of auditory threshold in adults and children with normal hearing and auditory neuropathy spectrum disorder. American Journal of Audiology, 30(1), 28-42. doi.org/10.1044/2020_AJA-20-00062
Gilley, P. M., Sharma, A., Dorman, M., & Martin, K. (2006). Abnormalities in central auditory maturation in children with language-based learning problems. Clinical Neurophysiology, 117(9), 1949-1956.
Glick, H., & Sharma, A. (2017). Cross-modal plasticity in developmental and age-related hearing loss: clinical implications. Hearing Research, 343, 191-201.
Glick, H. A., & Sharma, A. (2020). Cortical neuroplasticity and cognitive function in early-stage, mild-moderate hearing loss: Evidence of neurocognitive benefit from hearing aid use. Frontiers in Neuroscience, 14. doi: 10.3389/fnins.2020.00093
Sharma, A., Nash, A. A., & Dorman, M. (2009). Cortical development, plasticity and re-organization in children with cochlear implants. Journal of Communication Disorders, 42(4), 272-279.
Sharma, A., Glick, H., Campbell, J., & Biever, A. (2013). Central auditory development in children with hearing impairment: Clinical relevance of the P1 CAEP biomarker in children with multiple disabilities. Hearing, Balance and Communication, 11(3), 110-120.
Sharma, A., Campbell, J., & Cardon, G. (2015). Developmental and cross-modal plasticity in deafness: evidence from the P1 and N1 event related potentials in cochlear implanted children. International Journal of Psychophysiology, 95(2), 135-144.
Citation
Sharma, A. (2021). 20Q: Harnessing neuroplasticity in hearing loss for clinical decisionmaking. AudiologyOnline, Article 27826. Available at www.audiologyonline.com