From the Desk of Gus Mueller
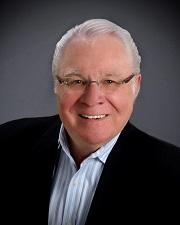
Tell me if you’ve heard this one before. Your Monday morning patient is in his 50s, has a history of some noise exposure (occasional hunting), and states that over the past few years he is starting to have trouble understanding conversations in background noise. He also has noticed some intermittent tinnitus. You do your standard audiologic battery, and find the pure-tone thresholds are normal (no thresholds worse than 15 dB HL), all immittance findings are normal, and his speech recognition (in quiet) is excellent.
Armed with these test results you need to sit down and talk to the fellow. What do you say? He’s a lucky man for having such good hearing in his 50s? But didn’t he say his hearing isn’t so good? Why is he saying this, you wonder? Troubles at home? Troubles at work? Shouldn’t the test results agree with the patient’s history?
Maybe not. Recent findings from animal studies of noise-induced and age-related hearing loss suggest that cochlear synapse loss is a likely contributor to difficulties understanding speech in noise, and may be an instigating factor in the generation of tinnitus and hyperacusis - even when hearing thresholds are normal. This cochlear synaptopathy may be widespread in acquired sensorineural hearing loss; it has been referred to as “hidden hearing loss” in that test results using the traditional audiologic battery often will be normal. While the dysfunction may be hidden to the audiologist, it’s very real for the patient.
We have one of the leading experts on the topic of hidden hearing loss with us this month at 20Q. Sharon G. Kujawa, Ph.D. is a clinician and an auditory neuroscientist. Dr. Kujawa is an Associate Professor of Otology and Laryngology, Harvard Medical School, and Director of Audiology Research and a Senior Scientist in the Eaton-Peabody Laboratories, Massachusetts Eye and Ear Infirmary in Boston. She also serves on the faculty of the Program in Speech and Hearing Biosciences and Technology at Harvard University.
Dr. Kujawa has numerous publications, most of which are in our field’s most prestigious journals. She has served on the executive board of both the AAA and the AAS, and the editorial board of several journals. She has received the AAA Distinguished Achievement Award. Sharon and her colleagues at the Mass Eye and Ear were recently awarded a Clinical Research Center Grant (P50) from the NIH/NIDCD to support work that aims to clarify the prevalence of cochlear synaptopathy, assess its consequences to supra-threshold sound processing and to identify non-invasive diagnostic markers to the underlying loss.
The term “hidden hearing loss” has been tossed around a lot in recent years, maybe not always with exactly the correct meaning. We’re fortunate to have a cutting-edge researcher on the topic here at 20Q to summarize and clarify the science behind the term.
Gus Mueller, PhD
Contributing Editor
August 2017
Browse the complete collection of 20Q with Gus Mueller CEU articles at www.audiologyonline.com/20Q
20Q: Cochlear Synaptopathy - Interrupting Communication from Ear to Brain
Learning Outcomes
After this course, readers will be able to:
- Explain the function of normal cochlear synapses, define synaptopathy and list some of its causes.
- Discuss synaptopathy in relation to: hair cell loss; DPOAEs; and audiometric thresholds.
- Describe what is meant by the term hidden hearing loss.
- Discuss synaptopathy research in humans as compared to animal models, including challenges and limitations in translating findings to clinical procedures, as well as future directions.
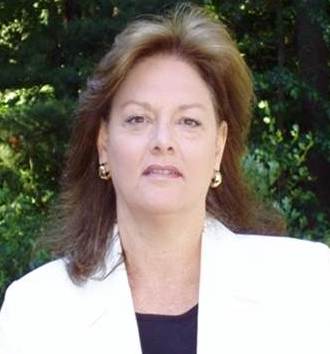
Sharon Kujawa
1. Bring me up to speed – what are cochlear synapses and what do they do?
In general terms, synapses are conduits for information flow. The cochlear ribbon-type synapses that are the focus of our recent research, are highly-specialized structures that support the transfer of information from inner hair cells (IHCs) to type I auditory nerve fibers (ANFs) and ultimately, to the brain. Here, the synapse is made up of a pre-synaptic ‘ribbon’ with its associated neurotransmitter-containing vesicles on the IHC side and post-synaptic receptors for the neurotransmitter on the nerve fiber terminal side. We are fortunate that powerful immunostaining and imaging techniques exist that allow us to ‘see’ these structures of interest (Figure 1). With these tools in hand, we can study such structures in normal ears and determine when and how they are altered with age, disease or insult.
IHC synapses with afferent nerve fibers contain the cellular machinery to support fast and precise signaling over sustained periods of time, graded over a broad dynamic range. These features of cochlear synaptic transmission allow a faithful representation of information that was contained in the original stimulus to be coded in the neural response. There are excellent reviews of the structure and remarkable capabilities of these ribbon synapses for those of you interested in greater detail (Matthews & Fuchs, 2010).
Figure 1. Left: Schematic of an IHC with 2 of its ~20 auditory nerve fibers. One synapse is circled, showing a pre-synaptic ribbon (red) and post-synaptic fiber terminal (green). Right: Immunostained tissue from a normal ear showing a row of IHC nuclei and associated synaptic and neural elements. One synapse is circled, the image magnified below, showing a pre-synaptic ribbon and post-synaptic terminal. After Kujawa and Liberman, 2009.
2. The ‘synaptopathy’ means that they can be lost?
Right. Cochlear synapses can be lost from noise exposure and aging, or their function can be altered or disabled due to genetic mutation. There are mutations that compromise the normal positioning of the pre-synaptic ribbons in apposition to their post-synaptic receptors, mutations that prevent the replenishment and fusion of vesicles or the packaging and release of their neurotransmitter. Each of these interferes with IHC communication with afferent nerve fibers, some preventing it altogether. Some of these mutations are responsible for hearing loss in humans; for example, DFNB9, DFNA25 (Moser, Predoehl, & Starr, 2013).
In our own laboratories, we’ve shown that synapses are lost rapidly after noise exposure and gradually with aging (Fernandez, Jeffers, Lall, Liberman, & Kujawa, 2015; Kujawa & Liberman, 2009; Lin, Furman, Kujawa, & Liberman, 2011; Sergeyenko, Lall, Liberman, & Kujawa, 2013). In addition to these two very common etiologies of sensorineural hearing loss (SNHL) in humans, evidence is accumulating that synapses can be lost with exposure to the ototoxic and vestibulotoxic drug gentamicin, one of the most common antibiotics in use worldwide. Here, too, the primary targets appear to be the IHC – afferent fiber synapses (Ruan et al., 2013). For each of these SNHL etiologies, loss of IHC-to-afferent fiber communication, rather than loss of the hair cells themselves, comes first. Ultimately, we expect to find that this loss of synapses, and the nerve fiber loss that routinely follows, is pretty common in various forms of acquired SNHL.
3. Can we focus for a minute on the noise-induced loss of cochlear synapses?
Sure. Our initial studies showed that, when noise levels are set to produce a large, but reversible threshold shift, there is a large, acute loss of cochlear nerve synapses with IHCs. This can occur without any loss of hair cells. This work focused on temporary threshold shift (TTS)-producing exposures, delivered to young adult mice from a normally-aging inbred strain (CBA/CaJ) to simplify the study of our main experimental questions. We’ve since documented noise-induced synapse loss in multiple mammalian species, including animals with lower frequency hearing more like humans, and for a broad range of TTS- and permanent threshold shift (PTS)-producing noise exposures.
We know that synapse loss after noise is very rapid; up to about 40-50% loss can be seen within an hour or so of exposure. We know that this loss is followed by loss of the affected type 1 fibers, which is underway in days, and that it ultimately progresses to loss of the cell bodies of these neurons in the spiral ganglion. The ganglion cell loss is slow and can progress for a very long time; months to years in the mouse, and years to perhaps decades in humans; see Kujawa and Liberman (2009) for further discussion of this time course. Consequences to function, however, are immediate: Once the synapse dies, the communication between the sensory hair cell and the nerve fiber is interrupted, no matter how long it takes the ganglion cell to die.
4. That’s pretty scary; do all noise exposures kill synapses?
No, they don’t. We’ve identified a number of exposures, in several species, that produce TTS without synaptopathy. However, even in the mouse, which is the model best characterized with regard to synaptopathy after noise, we don’t fully understand why certain exposures do, and certain others do not, result in synaptic loss. Currently, the exposure characteristics required to produce (or not produce) synaptopathy in humans are completely uncharacterized.
5. What does this synapse loss do to function?
At this point, it might be helpful to remind you that the organization of the cochlear afferent innervation is such that about 95% of cochlear afferent fibers are associated with IHCs (Spoendlin, 1972). In the mouse, each of these type I fibers makes a single contact with a single IHC using a single synapse, as shown in the schematic of Figure 1. Functionally, each of these fibers contributes a tiny potential to the whole nerve response (Antoli-Candela & Kiang, 1978).
This arrangement makes IHC synaptopathy a key variable. When a synapse is lost, its associated nerve fiber is silenced. And, because of this 1-fiber to 1-IHC via 1-synapse innervation pattern, the magnitude of the neural response decline in ears with synaptopathy is proportional to the magnitude of the synapse loss (Sergeyenko et al., 2013). So, neural responses that capture the afferent outflow from the cochlea - for example, the auditory nerve compound action potential (CAP) or the wave I component of the ABR - reflect this proportional loss: A roughly 50% synapse loss due to noise or aging can be seen as a roughly 50% reduction in suprathreshold amplitude loss in relevant cochlear regions. In the case of our standard TTS-producing exposure, cochlear amplification was not permanently altered, so the OHC-based DPOAEs recorded in the same ears were present with normal thresholds and suprathreshold amplitudes. In such ears, the persistent neural decline is clearly revealed in the ABR wave 1 response (compare Figure 2 a versus b; Kujawa & Liberman, 2009). Beyond these comparisons of the functional integrity of OHCs and neurons, our labs and others are beginning to characterize responses to more complex signals, in quiet and in noise, including responses that reflect activity at levels beyond the periphery, and including perceptual consequences of synaptic loss.
Figure 2. Despite threshold recovery, synaptopathic ears show permanent declines in ABR (b), but not DPOAE (a) suprathreshold amplitudes. At 8 weeks post noise, suprathreshold amplitudes of ABR wave 1, the far-field response of the cochlear nerve, was less than half of the control values in regions where TTS was maximal. Shown are means + SE for CBA/CaJ mice exposed at 16 weeks. Source: Kujawa & Liberman, 2009.
6. You said that synapses disappear with aging, too. Is that simply because noise exposure can be a significant contributor to the changes we typically attribute to aging?
Yes and no. Noise-exposed ears age, and the patients you see in the clinic may present with an interactive combination of injury from noise and aging that is only partially reflected in their audiogram. These effects of noise and aging can be studied singly and in combination in the lab. When we do that, we see that aging, alone, is associated with a gradually progressive loss of synapses. The loss begins early, well before changes in thresholds or hair cell losses are evident in the same ears, and progresses gradually, throughout the cochlea and throughout the lifespan (Fernandez et al., 2015; Sergeyenko et al., 2013). We have characterized the noise environment of our animals in the animal care facility. The aging animals we study are born and raised in noise backgrounds that are unlikely to cause large synaptic loss. But, is it possible that a lifetime of moderate-level exposure could produce some synapse loss? Maybe; that outcome has been hypothesized (Maison, Usubuchi, & Liberman, 2013; Sergeyenko et al., 2013), but the relevant studies have not yet been done.
Although we have seen up to about 50% loss of synapses within hours of noise exposure, we have documented, in the same inbred strain of mouse, that it takes a lifetime (about 2.3 years) to lose a similar percent of these synapses in mice never intentionally exposed to noise. But, if you expose these guys to a TTS- and synaptopathy-producing noise as young adults, and then let them get old without additional exposure, their ears and hearing show declines with age that are exaggerated from those seen in animals aged without exposure - even when threshold shifts from the noise recovered in the short term (Fernandez et al., 2015).
Noise exposures producing TTS in humans likely occur far more commonly than exposures producing PTS. And, although TTS traditionally has not been considered of lasting significance due to recovered thresholds, our studies show that such exposures can have not only permanent, but progressive consequences for aging ears and hearing (Fernandez et al., 2015). Studying such outcomes in humans will be challenging, but of great clinical and public health significance.
7. Just how widespread is this cochlear synapse loss?
Loss of synapses with noise or aging has been seen in every mammal evaluated so far, including mice, rats, guinea pigs, gerbils, chinchillas and even in non-human primates (e.g., Fernandez et al., 2015; Hickox, Larsen, Heinz, Shinobu, & Whitton, 2017; Kujawa & Liberman, 2009; Lin et al., 2011; Sergeyenko et al., 2013; Valero et al., 2017). Importantly, synaptic and afferent terminal loss is present, and dramatic, in human temporal bones having no significant loss of inner or outer hair cells or other known otopathology (Viana et al., 2015). Just as in the mice we evaluated, the magnitude of this loss increased with age, and the synaptic and afferent terminal losses could be much greater than ganglion cell losses (Makary, Shin, Kujawa, Liberman, & Merchant, 2011). Together, these observations suggest that cochlear synapse loss in aging humans is initially primary, beginning before hair cells are lost, and the ganglion cell loss that follows does so slowly. So, both the audiogram and the ganglion cell counts will underestimate the degree of the deafferentation present in these aging human ears.
8. Are you saying that neural hearing loss is common rather than relatively rare? I always thought that the hair cells, especially the outer hair cells, were the primary targets of exposures like noise.
Most adult hearing impairment is sensorineural in nature and arises from inner ear dysfunction. The field has long held that hair cells, particularly the OHCs, are the most vulnerable cochlear elements, with their injury or loss giving rise to the sensory component of sensorineural hearing loss. We have further believed that cochlear neural loss commonly occurs only after, and because of, IHC degeneration. This view of hair cells as the primary targets in the inner ear and the loss of neurons as a secondary consequence of that loss has dramatically shaped approaches to diagnosis, to treatment and to prevention of hearing loss – specifically, to prevention of threshold shifts.
Work from our lab and others over the last ~10 years, has shown that this sequence of events is not true for key causes of acquired sensorineural hearing loss, including noise, aging, and certain ototoxic drugs (Liberman & Kujawa, 2017). These insults can cause massive loss of cochlear synapses without any hair cell loss. In revealing these outcomes, this work has shifted some of the focus back onto the neural component of sensorineural, which appears to be very common, indeed.
9. You mention ears with massive synaptic and neural losses, but normal thresholds. How can so many of them die without affecting threshold sensitivity?
Great question! Several observations led us to suspect that our experimental manipulations were targeting just a subset of auditory neurons. For a range of noise doses, and in an age-graded series of mice, we recorded synapse losses that reached a maximum of about 40-50%. And, we had the additional observation that this dramatic loss of synapses could be seen in ears with completely recovered threshold sensitivity. How to reconcile these findings?
We subsequently performed some single unit recordings that shed light on this issue. These experiments revealed that post-noise synaptic and neural losses were initially dominated by the subset of cochlear nerve fibers with high thresholds and low spontaneous rates (SRs) of firing (Furman, Kujawa, & Liberman, 2013). These low-SR fibers make up about 40% of the total population of cochlear nerve fibers that communicate with the IHCs. By virtue of their relatively high thresholds, they don’t contribute to threshold detection in the normal ear, so their loss doesn’t elevate thresholds. It is interesting in this regard, that a study of cochlear nerve activity in the aging gerbil had previously reported a primary loss of low-SR fibers in the oldest animals (Schmiedt, Mills, & Boettcher, 1996).
10. I have more trouble understanding conversations in noisy situations than I used to when I was younger, but my audiometric thresholds are still good. Could this be related to the fibers you’re discussing?
Very possibly. These same low-SR fibers I was just talking about are particularly resistant to continuous noise masking, making them important in coding responses to transient signals in the presence of background noise (Costalupes, Young, & Gibson, 1984). This preferential low-SR fiber loss in noise and aging, then, has suggested to our group and others that the synaptopathy should contribute to speech-in-noise difficulties, even before thresholds are elevated.
The literature offers no shortage of reports, supported by the near ubiquity of personal experience (Ruggles, Bharadwaj, & Shinn-Cunningham, 2012), that speech understanding becomes poorer, particularly in noisy or other difficult environments, and performance on various suprathreshold tasks declines, in ways that are not well explained by changes in threshold sensitivity. These performance declines are evident by middle age (Grose, Hall, & Buss, 2006) but may be progressive throughout the adult life span (Snell & Frisina, 2000). More recently, studies of humans with normal audiograms but higher noise exposure risk profiles (Bramhall, Konrad-Martin, McMillan, & Griest, 2017; Liberman, Epstein, Cleveland, Wang, & Maison, 2016) have shown reduced ABR wave I amplitudes, consistent with those seen in the mice with synaptopathy, and deficits in suprathreshold temporal processing, in performance on speech-in-noise tests and abnormal auditory perceptions like tinnitus (Bharadwaj, Masud, Mehraei, Verhulst, & Shinn-Cunningham, 2015; Gu, Halpin, Nam, Levine, & Melcher, 2010; Schaette & McAlpine, 2011). Interestingly, the subjects studied by Liberman et al. (2016) also displayed symptoms of heightened sound annoyance and avoidance, suggesting a possible role for cochlear neurodegeneration in the development of hyperacusis.
11. Cochlear neurodegeneration can play a role in tinnitus and hyperacusis, too?
We know that noise damage causing only temporary elevations in audiometric thresholds can be associated with permanent perceptual anomalies like tinnitus or hyperacusis, and it is possible that cochlear synaptopathy/peripheral deafferentation is a key instigator of the changes that ultimately result in these abnormal perceptions (Bauer, Brozoski, & Myers, 2007; Hickox & Liberman, 2014). Here, the notion is that gain in auditory central circuits is increased when neural signals from the periphery are reduced (Chambers et al., 2016; Kaltenbach & Afman, 2000). Mice with normal thresholds but noise-induced synaptopathy and reduced ABR wave 1 amplitude, reflecting reduced afferent outflow from the cochlea, showed elevated startle responses (Hickox & Liberman, 2014). Humans with normal thresholds and symptoms of tinnitus and/or reduced sound-level tolerance, also tended to have reduced wave I, but enhanced wave V amplitudes, compared with individuals without tinnitus, that had matched, normal audiograms (Gu et al., 2010; Schaette & McAlpine, 2011). Again, this finding may reflect central gain adjustments in response to the reduced neural activity in the periphery. Findings in humans are inconsistent, however; a recent study failed to find evidence of reduced ABR wave I or EFR alterations in humans with subjective report of tinnitus (Guest, Munro, Prendergast, Howe, & Plack, 2017).
12. In recent years I’ve heard the term ‘hidden hearing loss’ tossed around a lot. Does this have anything to do with what you’re talking about?
Yes. We’ve known for a long time that audiometric thresholds and otopathology are not always well aligned and problems with speech in noise do not necessarily track with threshold sensitivity (Dubno, Dirks, & Morgan, 1984). Our work has shed new light on this disconnect, showing that cochlear synapses are highly vulnerable to noise and aging, and that even dramatic synaptopathy can hide behind a normal audiogram. This noise-induced or age-related cochlear synaptopathy has been termed “hidden hearing loss” (Schaette & McAlpine, 2011), because the kind of subtotal synapse loss we’ve been talking about (or IHC loss, for that matter) doesn’t cause elevation of electrophysiological or behavioral thresholds until it’s about 80 – 90% complete (Lobarinas, Salvi, & Ding, 2016; Schuknecht & Woellner, 1955). It has also been hidden because the loss of synapses is not visible in routine histological material and because the cell bodies of the affected cochlear neurons, which are easy to see in this material, survive for years to decades despite their loss of functional connection to the hair cells. To reveal the synaptopathy, we developed sensitive immunostaining techniques and quantification strategies for pre- and post-synaptic markers in the IHC area (Figure 1) and studied the histopathology relative to changes in function.
Normal thresholds or not, this loss of IHC – neural communication is expected to have significant perceptual consequences. And, if these consequences are as we hypothesized back in 2009, the resulting hearing loss (read: reduced function rather than threshold elevation) won’t be hidden at all, as Kraus and White-Schwoch (2016) clearly point out in their recent article.
A review of a series of experiments by Salvi and colleagues also makes this point clearly (Salvi et al., 2017). Chinchillas given the anti-cancer drug carboplatin can display massive and quite selective IHC/type I fiber loss with normal detection thresholds in quiet and normal DPOAEs. In noise, however, behavioral thresholds are elevated and remote masking of tones is exaggerated. Sound-evoked responses recorded at the level of the auditory cortex are larger than in untreated animals, suggesting a central gain adjustment in response to the peripheral deafferentation. The authors offer that, while this gain may allow auditory system compensation for such peripheral insult in quiet, it is not sufficient to improve performance in difficult listening conditions and may set the stage for an excessive gain-instigated hyperacusis.
13. Seems to me that there are other forms of hearing dysfunction that aren’t necessarily revealed by the pure tone audiogram?
True. The kind of subtotal failure of synaptic communication we’ve discussed here results in functional declines that are not revealed in the threshold audiogram. In reality, there could be multiple paths to this audiometric outcome, right? For example, we’ve just mentioned the case where there is a failure at the level of the IHCs that results in subtotal loss or injury to these sensory cells due to carboplatin, and we’ve mentioned genetically-based synaptopathies, neuropathies and insults like noise. All of these involve peripheral sites of damage. Beyond the periphery, and in addition to the central consequences of these peripheral injuries, there is an extensive literature that points to pathologies of more central origin that can be associated with auditory performance declines poorly reflected in thresholds.
14. The animal studies you mention are very convincing; do we know whether cochlear synaptopathy is a big deal in humans?
Given that noise- and age-related cochlear synaptopathy has been seen in every animal model studied, it is difficult to imagine that humans will be fundamentally different in this regard. Motivated by the very clear findings in the animal models, investigators are trying to clarify the extent to which cochlear synaptopathy is present...and a problem in humans. From preliminary study of human temporal bones (Viana et al., 2015), we know that dramatic degeneration of synapses and peripheral axons of auditory nerve fibers can be present and age-progressive in human ears without significant hair cell loss in the same regions. There is prior work as well, also in human temporal bones, that points to loss of peripheral terminals at the IHCs with age and after aminoglycoside exposure (reviewed in Viana et al., 2015). At present, this approach is our only direct assessment of cochlear synaptic and neural losses in humans.
15. You had the easy job; you noise-exposed mice, tested their hearing, and then counted their synapses and hair cells so you could see how the noise-induced synapse loss changed their hearing function. How will I diagnose cochlear synaptopathy in my patients without this kind of access to the underlying injury?
Beyond temporal bones, we won’t have direct knowledge of underlying injury in human ears, as you note. Our approach has been to perform the experiments in animal models of human hearing loss, studying function largely using techniques that can be applied non-invasively in humans, and then looking at the histological changes in the same ears. In synaptopathic mice and in humans high risk for such injury, IHC summating potentials (SPs) and suprathreshold cochlear nerve responses captured by CAP (ECoG in humans), ABR wave I and FFR approaches can reflect reduced neural activity when thresholds are normal, as we have discussed. A problem, of course, is that diagnosis of synaptopathy in ears with threshold sensitivity loss is more challenging since reduced cochlear amplifier function can also reduce neural response amplitudes. In addition, amplitudes of such responses in humans will be more variable than among genetically-identical mice, even for individuals with normal threshold audiograms. Recognizing these challenges, we and other groups working on this problem hope to devise a battery of physiological and psychophysical tests that are robust to these challenges to diagnose the presence of this underlying injury.
16. Is this a test battery that would be feasible in a busy clinical setting?
That’s a primary goal! Any clinician following this work realizes that some of the tests that have been so successful in diagnosing hidden hearing loss in the animal models would be time consuming, labor intensive and expensive to implement in the clinic. Part of the focus of our work going forward is to refine and focus these tests and others, to identify those key tools that are both clinically feasible and sensitive to neural pathology at early stages of auditory processing. These tools also will need to be robust to the greater variability that will be present in humans and will need to be informative even when sensory loss co-exists in the same ear; we all have our work cut out for us!
17. And what’s the role of the audiogram in all of this?
Noise and other cochlear insults can irreversibly damage outer hair cells (OHCs), which serve as the biological motors that amplify cochlear sound-evoked vibrations. Hair cell damage and loss are commonly reflected in elevated thresholds, and in humans, the behavioral pure tone audiogram is a key tool to help describe this overt hearing loss. The threshold audiogram provides information relative to the magnitude of the audibility loss, its pattern as a function of frequency, and to some extent underlying site(s) of lesion (e.g. middle ear, inner ear). We use the audiogram with the realization that it provides important information, but is not a perfect reflection of the health or the pathology of the auditory system.
We now appreciate that the threshold audiogram (and electrophysiologic and otoacoustic response thresholds for that matter) is silent to diffuse synaptopathy and the subtotal neurodegeneration that follows. In the example given in Figure 3, a 63-year-old male with a noise exposure history showed, in life, an audiometric threshold loss with a notched configuration of the type commonly associated with such exposure. Not unexpectedly, analysis of the temporal bones showed widespread OHC loss at the corresponding basal cochlear frequencies. In the same ear, there is virtually no IHC loss, but very significant loss of the peripheral processes of cochlear neurons. This primary neural loss, co-existing with OHC loss, results in a ‘mixed’ sensory + neural loss.
Figure 3. Audiometric thresholds and cochlear histopathology (black = missing cells) for the left ear of an individual with reported occupational history of noise exposure and bilateral noise induced hearing loss. Significant OHC loss in the cochlear basal turn, well-preserved IHCs, but primary neural loss (peripheral fiber but no ganglion cell loss yet) in the same cochlear region. Source: Mass Eye and Ear Temporal Bone Image Library.
As this case reveals, common causes of hearing loss in humans - aging, exposure to noise or ototoxic drugs - may sometimes produce complex mixtures of sensory and neural pathology. The audiogram will continue to be a key element of these evaluations. Beyond standard testing, we also know that threshold sensitivity at extended high frequencies can provide evidence of injury, even when thresholds at standard test frequencies are normal; for example, with age (Dubno, Horwitz, & Ahlstrom, 2003), from ototoxic drugs (Fausti et al., 1994) and from noise (Bramhall et al., 2017; Liberman et al., 2016). It is also possible that the degree of currently hidden underlying injury may help explain why different people with similar audiograms can have very different perceptual difficulties, and different benefit from amplification devices.
18. What are the implications of noised-induced synaptopathy for federal exposure regulations?
The implications are significant. Noise exposure regulations consider noise risk primarily in terms of PTS. We and others have provided clear evidence, in multiple species, that synapse loss begins at noise doses insufficient to produce PTS. It is almost certain that humans will not provide an exception to this general observation.
However, we also know that different species show different vulnerabilities - to noise-induced hair cell loss, to threshold shifts and now, to synapse loss. We don’t currently know where ‘safe’ and ‘not safe’ fall in terms of noise or other ototoxic exposures giving rise to cochlear synapse loss in humans. But, since preserving thresholds is not the same as preserving function, this growing body of evidence suggests that it is time to revisit our assumptions and assessments.
19. Let’s say all of the labs working on this come up with some nice diagnostic tools for me. What will I do with this information? How will it change the way I manage my patients?
By clarifying true functional consequences of exposure, of aging, such information may help validate the patient’s complaint and the family’s observations. Everyone has evaluated patients with perceptual complaints that don’t appear to align very well with their clinical test findings. We know that ‘normal hearing’ and ‘normal thresholds’ are not the same thing. Yet, when communicating the results of our assessments, we often appear to equate the two, telling the patient with normal thresholds and normal speech in quiet that their hearing is normal. To the patient, this may sound like a dismissal of their difficulties. At the very least, it does little to address the reason for their visit and for their perceived decline in hearing function. Hopefully, these highly-anticipated diagnostic tools will better inform such conversations.
Access to such information also should improve early identification, supporting prevention of injury. From occupational hearing conservation programs to hospital ototoxic drug monitoring protocols, the current standard of care is to monitor audiometric thresholds, sometimes to extended high frequencies and sometimes coupled with otoacoustic emissions, to provide early warning of injury. If synaptopathy in humans precedes hair cell death or dysfunction from these insults, as it does in the animal models, our current strategies are not doing a good job of preventing hearing impairment. Given that even massive cochlear synaptopathy can be hidden in a normal threshold audiogram, it is instead possible that we are allowing significant cochlear pathology to silently accumulate in many individuals.
Improved diagnosis will inform our selection of appropriate rehabilitative/treatment strategies and will provide a means to assess treatment efficacy. Beyond the importance of understanding the mechanisms underlying the perceptual impairments of SNHL in general, and cochlear synaptopathy in particular, refinement of our assessments is needed as regenerative therapies become a more realistic goal. IHCs and ganglion cell bodies of the afferent neurons can live a long time after the synapses that connect them are gone. This means that there may be a long window of opportunity to apply regenerative treatments that could reconnect them (Sly et al., 2016; Suzuki, Corfas, & Liberman, 2016). As clinical trials become possible, accurate diagnosis will be essential to identify candidates for the treatments and to assess outcomes.
20. What’s next?
We believe that cochlear synaptopathy is widespread in humans, whether ‘hearing loss’ is evident on the threshold audiogram or not, and that the degree of synaptopathy can be an important determinant of the overall functional impairment. Having said that, we need to better understand the prevalence and nature of cochlear synaptopathy in humans. What causes it and when? What are its consequences for suprathreshold sound processing, for tinnitus and hyperacusis? We need to identify diagnostic markers that can non-invasively, sensitively and efficiently reveal its presence in the clinical setting. We need to develop therapies to support synaptogenesis and neural sprouting, with the goal of reconnecting functioning sensory cells and neurons. A large, existing literature tells us that peripheral injury can have central consequences. Using our various models of cochlear synaptopathy, and extending the work to humans, an important goal is to characterize such outcomes.
These are early days and there are many unanswered questions. Let’s talk again with another 20Q down the road!
Acknowledgments
Work summarized here has been supported by grants from the NIH/NIDCD (R01 DC08577, R01 DC0188, and P30 DC05209), the Department of Defense (W81XWH-15-1-0103) and the Office of Naval Research (N00014-16-1-2867).
References
Antoli-Candela, Jr F. & Kiang, N.Y.S. (1978). Unit activity underlying the N1 potential. In: RF Naunton, C. Fernandez (Eds.), Evoked electrical activity in the auditory nervous system. NY: Academic Press, New York. pp. 165-191.
Bauer, C.A., Brozoski, T.J., & Myers, K. (2007). Primary afferent dendrite degeneration as a cause of tinnitus. Journal of Neuroscience Research, 85(7), 1489-1498.
Bharadwaj, H.M., Masud, S., Mehraei, G., Verhulst, S., & Shinn-Cunningham, B.G. (2015). Individual differences reveal correlates of hidden hearing deficits. Journal of Neuroscience 35(5), 2161-2172.
Bramhall, N.F., Konrad-Martin, D., McMillan, G.P., & Griest, S.E. (2017). Auditory brainstem response altered in humans with noise exposure despite normal outer hair cell function. Ear and Hearing, 38(1), e1-e12.
Chambers, A.R., Resnik, J., Yuan, Y., Whitton, J.P., Edge, A.S., Liberman, M.C., & Polley, D.B. (2016). Central gain restores rate-based auditory processing following near-complete cochlear denervation. Neuron, 89, 1-13.
Costalupes, J.A., Young, E.D., & Gibson, D.J. (1984). Effects of continuous noise backgrounds on rate response of auditory nerve fibers in cat. Journal of Neurophysiology, 51(6), 1326-1344.
Dubno, J.R., Dirks, D.D., & Morgan, D.E. (1984). Effects of age and mild hearing loss on speech recognition in noise. Journal of the Acoustical Society of America, 76(1), 87-96.
Dubno, J.R., Horwitz, A.R., & Ahlstrom, J.B. (2003). Recovery from prior stimulation: Masking of speech by interrupted noise for younger and older adults with normal hearing. Journal of the Acoustical Society of America, 113(4 Pt 1), 2084-2094.
Fausti, S.A., Larson, V.D., Noffsinger, D., Wilson, R.H., Phillips, D.S., & Fowler, C.G. (1994). High-frequency audiometric monitoring strategies for early detection of ototoxicity. Ear and Hearing, 15(3), 232-239.
Fernandez, K.A., Jeffers, P.W., Lall, K., Liberman, M.C., & Kujawa, S.G. (2015). Aging after noise exposure: acceleration of cochlear synaptopathy in “recovered” ears. Journal of Neuroscience, 35(19), 7509-7520.
Furman, A.C., Kujawa, S.G., & Liberman, M.C. (2013). Noise-induced cochlear neuropathy is selective for fibers with low spontaneous rates. Journal of Neurophysiology, 110(3), 577-586.
Grose, J.H., Hall, J.W. III, & Buss, E. (2006). Temporal processing deficits in the pre-senescent auditory system. Journal of the Acoustical Society of America, 119(4), 2305–2315.
Gu, J.W., Halpin, C.F., Nam, E.C., Levine, R.A., & Melcher, J.R. (2010). Tinnitus, diminished sound-level tolerance, and elevated auditory activity in humans with clinically normal hearing sensitivity. Journal of Neurophysiology, 104(6), 3361-3370.
Guest, H., Munro, K.J., Prendergast, G., Howe, S., & Plack, C.J. (2017). Tinnitus with a normal audiogram: Relation to noise exposure but no evidence for cochlear synaptopathy. Hearing Research, 344, 265-274.
Hickox, A.E., & Liberman, M.C. (2014). Is noise-induced cochlear neuropathy key to the generation of hyperacusis or tinnitus? Journal of Neurophysiology, 111(3), 552-564.
Hickox, A.E., Larsen, E., Heinz, M.G., Shinobu, L., & Whitton, J.P. (2017). Translational issues in cochlear synaptopathy. Hearing Research, 349, 164-171.
Kaltenbach, J.A., & Afman, C.E. (2000). Hyperactivity in the dorsal cochlear nucleus after intense sound exposure and its resemblance to tone-evoked activity: a physiological model for tinnitus. Hearing Research, 140(1-2), 165-172.
Kraus, N., & White-Schwoch, T. (2016). Not-so-hidden hearing loss. Hearing Journal, 69(5), 38,40.
Kujawa, S.G., & Liberman, M.C. (2009). Adding insult to injury: cochlear nerve degeneration after “temporary” noise-induced hearing loss. Journal of Neuroscience 29(45), 14077–14085.
Liberman, M.C., Epstein, M.J., Cleveland, S.S., Wang, H., & Maison, S.F. (2016). Toward a differential diagnosis of hidden hearing loss in humans. PLoS One, 11(9), e0162726.
Liberman, M.C., & Kujawa, S.G. (2017). Cochlear synaptopathy in acquired sensorineural hearing loss: manifestations and mechanisms. Hearing Research, 349, 138-147.
Lin, H.W., Furman, A.C., Kujawa, S.G., & Liberman, M.C. (2011). Primary neural degeneration in the Guinea pig cochlea after reversible noise-induced threshold shift. Journal of the Association for Research in Otolaryngology, 12(5), 605-616.
Lobarinas, E.R., Salvi, R., & Ding, D. (2016). Selective inner hair cell dysfunction in chinchillas impairs hearing-in-noise in the absence of outer hair cell loss. Journal of the Association for Research in Otolaryngology, 17(2), 89-101.
Maison, S.F., Usubuchi, H., & Liberman, M.C. (2013). Efferent feedback minimizes cochlear neuropathy from moderate noise exposure. Journal of Neuroscience, 33(13), 5542–5552.
Makary, C.A., Shin, J., Kujawa, S.G., Liberman, M.C., & Merchant, S.N. (2011). Age-related primary cochlear neuronal degeneration in human temporal bones. Journal of the Association for Research in Otolaryngology, 12, 711–717.
Matthews, G., & Fuchs, P.A. (2010). The diverse roles of ribbon synapses in sensory neurotransduction. Nature Reviews Neuroscience, 11(12), 812-22. doi: 10.1038/nrn2924.
Moser, T., Predoehl, F., & Starr, A. (2013). Review of hair cell synapse defects in sensorineural hearing impairment. Otology Neurotology, 34(6), 995-1004.
Ruan, Q., Ao, H., He, J., Chen, Z., Yu, Z., Zhang, R., Wang, J., & Yin, S. (2014). Topographic and quantitative evaluation of gentamicin-induced damage to peripheral innervation of mouse cochleae. NeuroToxicology, 40, 86-96.
Ruggles, D., Bharadwaj, H., & Shinn-Cunningham, B.G. (2012). Why middle-aged listeners have trouble hearing in everyday settings. Current Biology, 22(15), 1417-1422.
Salvi, R., Sun, W., Ding, D., Chen, G.D., Lobarinas, E., Wang, J.,…Auerback, B.D. (2017). Inner hair cell loss disrupts hearing and cochlear function leading to sensory deprivation and enhanced central auditory gain. Frontiers in Neuroscience, 10, 621.
Schaette, R., & McAlpine, D. (2011). Tinnitus with a normal audiogram: physiological evidence for hidden hearing loss and computational model. Journal of Neuroscience, 31(38), 13452-13457.
Schmiedt, R.A., Mills, J.H., & Boettcher, F.A. (1996). Age-related loss of activity of auditory-nerve fibers. Journal of Neurophysiology, 76(4), 2799-2803.
Schuknecht, H.F., & Woellner, R.C. (1955). An experimental and clinical study of deafness from lesions of the cochlear nerve. Journal of Laryngology and Otology, 69(2), 75-97.
Sergeyenko, Y., Lall, K., Liberman, M.C., & Kujawa, S.G. (2013). Age-related cochlear synaptopathy: an early-onset contributor to auditory functional decline. Journal of Neuroscience, 33(34), 13686-13694.
Sly, D.J., Campbell, L., Uschakov, A., Saief, S.T., Lam, M., & O’Leary, S.J. (2016). Applying neurotrophins to the round window rescues auditory function and reduces inner hair cell synaptopathy after noise-induced hearing loss. Otology Neurotology, 37(9), 1223-1230.
Snell, K.B., & Frisina, D.R. (2000). Relationships among age-related differences in gap detection and word recognition. Journal of the Acoustical Society of America, 107(3), 1615-1626.
Spoendlin, H. (1972). Innervation densities of the cochlea. Acta-Otolaryngologica, 73, 235-248.
Suzuki, J., Corfas, G., & Libeman, M.C. (2016). Round-window delivery of neurotrophin 3 regenerates cochlear synapses after acoustic overexposure. Scientific Reports, 6, 24907. doi: 10.1038/srep24907
Valero, M.D., Burton, J.A., Hauser, S.N., Hackett, T.A., Ramachandran, R., & Liberman, M.C. (2017, July). Noise-induced cochlear synaptopathy in rhesus monkeys (Macaca mulatta). Hearing Research. doi: 10.1016/j.heares.2017.07.003
Viana, L.M., O’Malley, J.T., Burgess, B.J., Jones, D.D., Oliveira, C.A., Santos, F.,…Liberman M.C. (2015). Cochlear neuropathy in human presbycusis: Confocal analysis of hidden hearing loss in post-mortem tissue. Hearing Research, 327, 78-88.
Citation
Kujawa, S. (2017, August). 20Q: Cochlear synaptopathy - interrupting communication from ear to brain. AudiologyOnline, Article 20946. Retrieved from www.audiologyonline.com